The aim of this task is to coordinate and demonstrate field inter-comparison activities for FRM OCR.
D-190 Write a Technical Report (TR-8): “Protocols and Procedures for Field Inter -Comparisons of Fiducial Reference Measurement (FRM) Field Ocean Colour Radiometers (OCR) used for Satellite Validation”
To underpin the validation of satellite OCR, it essential that above and in water radiometers used to collect FRM’s, to ascertain the accuracy of Sentinel 2 & 3 products, are inter-compared to assess data consistency and characterise uncertainties between instruments. In the absence of such inter-comparisons, the use of a wide range of instruments, methods and laboratories may only add to the uncertainty in the accuracy of Sentinel 2 & 3 products. The primary data product in satellite ocean colour used to generate biogeochemical concentrations of chlorophyll a (Chl a) and total suspended matter (TSM) that are widely distributed to the user community for monitoring the marine environment, is the spectral remote sensing reflectance (Rrs) measured from the satellite sensor as the top of atmosphere radiance. Measurements of this parameter in situ are generally obtained through the deployment of in-water and above water optical measurement systems (OMS). OMS include fixed platforms, ships and tethered buoys. Within the numerous OMS that exist, the following may lead to uncertainties in measured Rrs that will ultimately affect the accuracy assessment of the satellite products:
1. A range of different OMS methods are deployed, including above water radiometry, underwater profiling, underwater measurements at fixed depths or combined above/underwater measurements from floating systems.
2. Different processing schemes are applied to the data from these systems.
3. A range of calibration sources and methods for the absolute radiometric calibration of field instruments are used.
Best practices on each of these steps to guide the generation of radiometric FRM’s will be established in this project. Verification of these procedures will be quantified through a series of inter-comparison exercises that will be conducted in a range of different environmental conditions and biogeochemical provinces relevant to the Sentinel-2 and 3 missions.
A growing body of literature on inter-comparisons between radiometers, deployed for satellite ocean colour validation, exists most of which reside in grey literature (Hooker et al. 2002a, b, Johnson et al. 1999; Meister et al. 2002; Moore et al. 2010; Tilstone et al. 2004; Zibordi et al. 2003) with the notable exception of studies conducted at the The Acqua Alta Oceanographic Tower during the ENVISAT mission (Zibordi et al. 2012). The lessons learnt from these previous field inter-comparisons are as follows:
- NASA SIRREX 1-8 (Hooker et al. 2002a, b; Johnson et al. 1999; Zibordi et al. 2003): The SIRREX inter- comparison focused on spectral radiance responsivity measurements using the plaques and sensor calibration or stability monitoring with portable field sources. These resulted in an improvement in uncertainties between the spectral irradiance lamps used for calibration from 8% to 1%. During SIRREX 5 comparison between radiometers were conducted at Little Seneca Lake in the US. Differences in remote sensing reflectance (Rrs) were found to be due to environmental conditions, the illumination geometry, and systematic effects associated with the derivation of the downwelling irradiance from the reflected plaque radiance, or problems with the radiometers. These were addressed in SIRREX 8 through the publication of new laboratory methods for characterizing irradiance sensors (Zibordi et al. 2003).
- NASA SIMRIC 1 & 2 (Meister et al. 2002). The purpose of the SIMRICs was to ensure a common radiometric scale among the calibration facilities that are engaged in calibrating in-situ radiometers used for ocean color related research. The end result was an updated document on calibration procedures and protocols. Using these protocols during SIMRIC-2, the SeaWiFS Transfer Radiometer (SXR-II) measured the calibration radiances at six wavelengths from 411nm to 777nm which was compared against measurements from ten other laboratories. The agreement between laboratories was within the combined uncertainties for all but two laboratories. The likely errors in these were identified in the NIST calibrations.
- MERIS MAVT PlymCal 1-3 (Tilstone et 2004): The expected error for radiometric calibrations is 2%. PlyMCal 1 & 2 performed a suite of inter-comparisons including above and in water radiometry. Bio-spherical, PR650, SATLANTIC, SIMBADA, SPMR, TACCS and TRIOS radiometers were compared and referenced to the same NIST lamp and plaque. Their performance was within +/- 2%, except for the TRIOS radiance sensors. The TRIOS instrument was re-calibrated several time against the reference standard, but the error was still out of the target range. Following PlymCal, PML and project partners worked closely with TRIOS to resolve this.
- MERIS MVT (Moore et 2010): A field campaign at a coastal site off South West Portugal determined the accuracy of atmospheric and in-water measurements using a hyper-spectral, SATLANTIC buoy radiometer with a tethered irradiance chain; and a band-pass radiometer of the same design. An overall error for the TACCSsystem in these waters was 5% where Kd was known and 7% where Kd was extrapolated.
- ARC MERIS MVT (Zibordi et 2012): Inter-comparisons of above water radiometers (SeaPRISM & TRIOS) and in water radiometers (WISPER & TACCS) were performed under near ideal deployment conditions at the Acqua Alta Oceanographic Tower in the northern Adriatic Sea. All sensors were inter-calibrated through absolute radiometric calibration with the same standards and methods. The spectral water leaving radiance (Lw), above-water downward irradiance (Ed0+) and remote sensing reflectance (Rrs) were compared. The relative difference in Rrs was between −1 and +6 %. The spectrally averaged values of absolute difference were 6% for the above-water systems and methods to 9% for the in water systems and methods. The good agreement between sensors was achievable because of the stability of the deployment platform used.
A review of inter-comparison procedures and protocols deployed during the NASA SeaWiFs and MODIS and MERIS missions will be conducted. The Technical Report-8 on “Protocols and Procedures for Field Inter-Comparisons” will be produced in collaboration and consultation with the optics group at the EU Joint Research Centre (Zibordi et al.), who were the primary contractor to conduct field inter-comparisons during the MERIS mission, and who will be a non- funded collaborator in the project and Istituto di Scienze Marine (ISMAR), Venice, Italy, who will collaborate on the logistics of the FICE-AAOT.
D-200 A dedicated FICE implementation plan (FICE-IP) will be produced to Plan and manage and document all aspects of the FICE.
The implementation plan will be designed based on previous work carried out under NASA SIRREX and SIMRIC and ESA MVT field inter-comparisons. Repetition of the errors reported in these previous inter-comparisons will be reduced by:
- Calibrating all sensors at the same reference laboratory using the same plaques and NIST calibration sources prior to the AAOT.
- Use of SeaPRISM (above-water) and WiSPER (in-water) as reference sensors.
- Reducing environmental effects including tilt and roll and illumination geometry by using the stable AAOT platform.
- Reducing environmental effects as a result of heterogeneous conditions by conducting continuous above-water radiometry measurements throughout daylight hours for a period of ~5 weeks.
Field Inter-Comparison Experiments (FICE) that deploy FRM OCR in the field side-by-side.
The FICE experiments will be conducted at two principal platforms:
A. The Acqua Alta Oceanographic Tower (AAOT),
B. The Atlantic Meridional Transect (AMT).
These have been chosen as they both have a long history of use for satellite ocean colour validation and development during recent NASA and ESA missions (e.g. O’Reilly et al. 1998; Zibordi et al. 2006). Both platforms offer excellent deployment conditions for above water, floating and underwater profiling systems (e.g. Hooker et al. 2005), provide a range of oceanographic conditions to characterise measurement uncertainties and allow a large number of measurements to be taken per day (Brewin et al. 2014; Zibordi et al. 2002). Good agreement between radiometric sensors was achieved at the AAOT under ARC MERIS MVT because of the stability of the platform and the near ideal deployment conditions experienced at its location. The use of multi- sensor and method comparisons at the AAOT and long track above water radiometric comparison on the AMT will provide the volume and quality of data for a stand-alone publication in potentially high impact factor journal.
A. FICE-AAOT
The Acqua Alta Oceanographic Tower in off the Gulf of Venice, Italy, in the northern Adriatic Sea is a purpose built steel tower with a platform containing an instrument house to facilitate the measurement of ocean properties under exceptionably stable conditions. The platform has a long history of optical measurements to support and validate both NASA and ESA ocean colour missions (Zibordi et al. 2006; 2009b). An autonomous OMS has been developed at the tower, the data from which are widely used and accessed by the ocean colour community for satellite validation (Zibordi et al. 2004b, 2009c). Both in- and above-water optical measurements at the AAOT are taken under near ideal conditions due to the stability of the platform which has a fixed geometry, the frequency of clear sky conditions, the relatively low sun zenith angles and moderate to low sea states that are experienced at the tower. The water type at the tower can vary from clear open sea to turbid coastal. The atmospheric conditions are mostly dominated by continental and occasionally maritime, aerosols. This variability offers the opportunity perform field inter-comparisons under a wide range of environmental conditions. Continuous data acquisition of above water radiometry coupled with field campaigns to characterise the in water optics makes this site unique in the ability to inter-compare an array of sensors and methods at any one time.
All optical sensors will be inter-calibrated against the same standards and methods (by NPL in Task 3) prior to the FICE. The data products listed in Table 4-4. will be inter-compared. Data analysis will be conducted on centre wavelengths for Sentinel 2 & 3 (400, 412, 442, 510, 560, 620, 665, 673, 681, 708 nm). Uncertainty budgets will be quantified for each system and method. – see below).
Table 4-4. FRM products that will be inter-compared during the FICE-AAOT and FICE-AMT.
Fiducial Reference Measurement Abbreviation Units
Above water Apparent Optical properties AOPs
Remote sensing reflectance Rrs= Lu/Ed sr-1
Normalised water leaving radiance nLw mW cm-1 μm-1 sr-1
In water Apparent Optical properties AOPs
Photosynthetically active radiation PAR E m-2 s-1
Attenuation coefficient Kd m-1
Euphotic depth Zeu m
Downwelling Irradiance Ed mW cm-1 m-1
Upwelling radiance Lu mW cm-1 m-1
Upwelling irradiance Eu mW cm-1 m-1
Quantifying differences between methods:
In-water radiometric continuous profiles are usually made by winch or freefall. The accuracy of the resulting radiometric products depends on the sampling depth interval and on the depth resolution (D’Alimonte et al., 2010; Zibordi et al. 2004a, 2012). In some environments (particularly coastal, case 2 waters), the heterogeneous nature of the optical properties, a high accuracy in in water radiometric products can only be determined by sampling close to the surface (e.g. TAACS in Table 4-3.) and / or by producing a large number of measurements per unit depth (Zibordi et al., 2004b) as long as the variance in sensor tilt resulting from waves and currents is eliminated or reduced.
In-water fixed-depth profiles are normally obtained from the deployment of optical sensors on buoys at fixed depths (e.g. Antoine et al. 2008) or from buoys with autonomous profiling systems (e.g. Laurenco et al. 2000; Zielinski et al. 2006). These OPMs are capable of measuring Lu and Ed simultaneous at multiple depths. Assuming that tilt is negligible, the accuracy of the radiometric products is a function of the fixed depths used for the optical sensors, the acquisition rate and duration of logging interval (Zibordi et al., 2009a; 2012).
Above-water methods use measurements of total radiance from above the sea (LT), sky radiance Li and above water Ed(0+) which are used to determine Lw. The measurement geometry is determined by the sea-viewing angle, the sky- viewing angle and the difference between sun and sensor azimuth angles (Deschamps et al., 2004; Hooker et al., 2004; Zibordi et al., 2004b). The accuracy of Lw is dependent on the degree to which sun glint can be minimized which is normally done by choosing suitable measurement geometries (nominally +135º viewing angle; Mobley, 1999). The application of statistical filtering schemes on LT (Hooker et al., 2002a; Zibordi et al., 2002), correction methods based on known reflectance properties of seawater in the near-infrared (Ruddick et al., 2006), or polarisers to directly reduce sky- and sun glint (Fougnie et al., 1999) can also be used to minimise glint effects.
Each of these factors in the different methods will be considered in the FICE and the uncertainties arising from them will be calculated.
B. FICE-AMT
The Atlantic Meridional Transect (AMT) has been operated by the Plymouth Marine Laboratory (PML) in collaboration with National Oceanography Centre (NOC) Southampton for the past two decades. The cruise is conducted between the UK and the sparsely sampled South Atlantic during the annual passage from October to November of a NERC ship (RRS James Clark Ross, RRS James Cook or RRS Discovery). The transect covers several ocean provinces where key physical and biogeochemical variables such as chlorophyll, primary production, nutrients, temperature, salinity and oxygen are measured. The stations sampled are principally in the North and South Atlantic Gyres, but also the productive waters of the Celtic Sea, Patagonian Shelf and Equatorial upwelling zone are visited, which therefore offers a wide range of variability in which to conduct FICE for the FRM4SOC.
There are few calibration / validation sites in the blue water oligotrophic gyres of the global oceans, because of the cost of accessing and maintaining measurement platforms in such remote locations. The NOAA moored buoy MOBY (off Hawaii) has been used during the US Sea viewing Wide-Field-of-view Sensor (SeaWiFS), Moderate-resolution Imaging Spectroradiometer (MODIS) and Visible Infrared Imaging Radiometer Suite (VIIRS) missions to provide vicarious calibration data to monitor and reference to satellite Level2 Reflectance (L2R) data. Both MOBY and BOUSSOLE (the CNRS, France optical moored buoy) provided this capability for MERIS, but there were few independent sites in deep blue, case 1 waters that are used for ocean colour validation. AMT therefore offers an excellent opportunity to conduct field inter-comparisons at these sites.
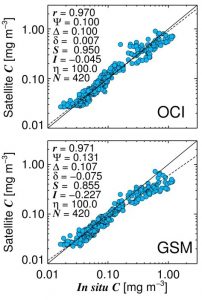
Figure 4-11. Comparison of two chlorophyll (denoted C) algorithms applied to ESA OC-CCI data with in situ chlorophyll on AMT19. Statistical tests used include: Pearson correlation coefficient (r); root-mean-square error (Ψ); unbiased root-mean-square error (Δ); absolute bias (δ); slope (S) and intercept (I) of a Type-2 regression; percentage of retrievals (η); and number of retrievals (N). All tests were performed on log10-transformed chlorophyll concentrations.
The AMT has an excellent heritage for ocean colour (OC) satellite calibration and validation. At IOC in 2014 and 2015, AMT was heralded as one of NASA SeaWiFS 10 greatest highlights and it was recommended this ocean observing platform be funded to provide vital calibration / validation data for future satellite missions. AMT not only provided vital FRM data for the duration of the SeaWiFS mission but also served as a developmental and inter-comparison platform for selecting the most accurate ocean colour algorithm for SeaWiFS. Many of the early AMTs in the late 1990s were financially supported by NASA for the early pre- and post-launch work on the SeaWiFS. This work included in-situ radiometric measurements to compare against the satellite derived values of water leaving radiance and coincidental measurements of chlorophyll for vicarious calibration and algorithm development. Recent AMTs have renewed the optical drive with continuous, highly accurate and well calibrated measurements of hyperspectral absorption, attenuation and backscatter (Inherent Optical Properties – IOPs) using an established optical flow-through set-up (WET Labs ECO-BB3 meter and WET Labs ACs; see Dall’Olmo et al. 2012) working from seawater from the ship’s clean flow- through system. Measurements of particulate absorption are calibrated with discrete HPLC chlorophyll measurements to derive continuous along-tack estimates of chlorophyll concentration (Brewin et al. 2014). This has resulted in unprecedented numbers of data points (e.g. 400 per cruise) for use in satellite validation work (e.g. Figure 4-11.).
PML have opportunistically taken coincident with hyperspectral radiometer measurements of water leaving radiance (SATLANTIC HYPERSAS; see Figure 4-12.).
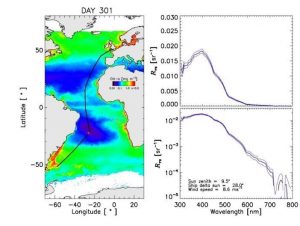
Figure 4-12. Hyperspectral remote-sensing reflectance (Rrs) data acquired using a HyperSAS radiometer at a station in the centre of the South Atlantic Gyre on AMT23.
The AMT-FICE will inter-compare the above-water measurements listed in Table 4-4., and the sensors and methods deployed by PML, JRC and RBINS (see Table 4-3.) along a 4000 mile transect in both productive and coastal waters as well as the clearest waters in the Atlantic Ocean and under Sentinel 2 and 3 swaths additionally allowing multi-sensor comparisons at the time of satellite match-ups. Uncertainty budgets on instrument calibration, measurement platform and measurement processing will be computed to ensure measurement traceability to NIST/NPL standards based on calibrations before, during and after the cruises. This will contribute to quantification of the errors in these FRMs and also in level 2 OLCI products in open ocean Atlantic environments.
The AMT-FICE will be conducted early in the project on AMT26 from 4 September to 11 October 2016 so that these initial ship-borne comparisons can be used to guide the main FICE at AAOT in 2017. The AMT-FICE will enable the consortium to develop knowledge of potential biases between measurements made by instruments under a range of operational and in water optical conditions. The AMT is costly and would be beyond the resources available to a single task and Lead within this ITT. PML will provide the ship time, personnel (both scientific, technical and ship) and additional data (CTD, biogeochemical concentrations, IOPs) at no cost to the project. Small resources are sought to cover the travel expenses of RBINS to and from the ship.
D-210 FICE Database (FICE-DB)
This task is the implementation of an open-access (via web page) database to store and retrieve FICE results from different experiments.
PML will implement a PostGIS database to store FICE results from the AAOT-FICE, and AMT-FICE. This open-source database software will be configured to store details of the instrument used in the AAOT-FICE, and AMT-FICE with additional ancillary data detailing it’s location, detector, observable properties, orientation, etc. using Open Geospatial Standards (OGC) Sensor Model Language (SensorML). The template will be similar to that shown in Figure 4-13.
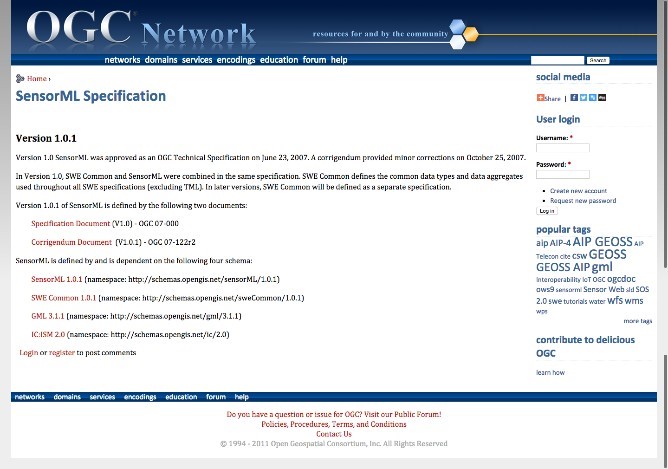
Figure 4-13. Template of Open Geospatial Standards (OGC) Sensor Model Language (SensorML) that will be used for FICE-DB.
The database would also be configured to record sensor observations or measurements, allowing multiple observable properties to be recorded for a single sensor. This database would be connected to two interfaces in order to facilitate the creation of sensor records and the subsequent addition of observations or measurements. A web interface will allow registered/logged in users to add new sensor information, and offer the ability to upload a correctly formatted CSV file of measurements, based on a provided and specified template, to populate the database. Upon successful upload, the file will be validated for format and content to ensure that only valid data records are added to the database. Once the record of a sensor has been created via the web interface it will then be possible to programmatically add sensor measurements using a clearly defined and documented Application Program Interface (API); this will allow continuing real-time, or near-real time, updates to be added to the database without direct user intervention. The database should be built architecture to ensure that can be moved between computers if necessary.
The database would also be connected to two interfaces for the retrieval and extraction of sensor measurements. A web interface would allow users, without having to be registered or logged in, to browse and search a list of sensors and observable properties that are available, and then to download the data for the selected sensor. The database interface shall allow users to visualise results from different FICE and analyse the performance of different field OCR FRM over time. The system will also be tested for potential future (beyond the life time of the project) data upload so that new results can be seamlessly be uploaded as they become available during the project. The second interface would offer programmatic access to the sensor records via OGC’s Sensor Observation Service (SOS), thus allowing users to make requests for sensor details and observations using their preferred scripting language. Providing access to the data via SOS means that sensor records can be included in external visualisation and/or analysis tools similar to those shown in Figure 4-14.
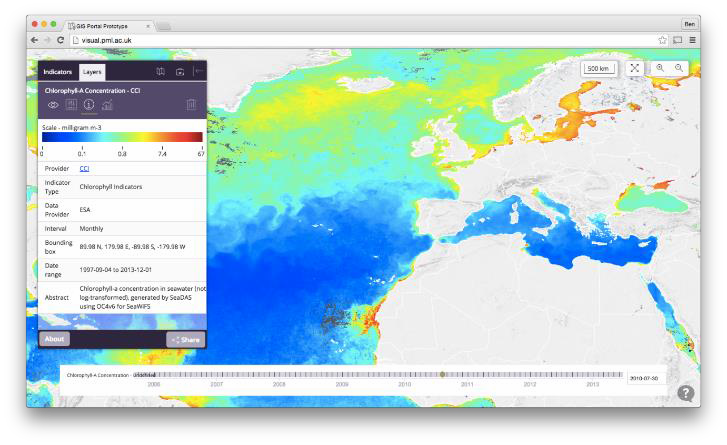
Figure 4-14. Example of GIS interface that will be used in the FICE-DB.
Processes for the systematic analysis and presentation
With the FICE results ingested to the PostGIS database it will be possible to perform match ups of the FICE results with Earth Observation (EO) data from Climate Change Initiative Ocean Colour data as well as data from Sentinel-2 and Sentinel-3 missions as they become available. A web based GIS tool will offer the ability to analyse and compare by extracting data from the FICE database using the SOS service access point and extracting relevant EO observations using OGC’s Web Map Service (WMS) and Web Coverage Service (WCS). Users will have the ability to create time series or scatter plots very quickly without having to download vast quantities of data.
D-220 Write a Technical Report (TR-9): “Results from the First FRM4S OC Field Inter-Comparison Experiment (FICE) of Ocean Colour Radiometers”
The technical report will include results from the field inter-comparison experiments at both the AAOT and AMT and will consider the following:
- Comparison of above-water radiometers (e.g. SeaPRISM, SATLANTIC, TRIOS RAMSES) under near ideal conditions in open ocean and turbid coastal with predominantly continental aerosols; in the North and South Atlantic Gyres, Shelf waters of the Celtic Sea and Patagonia and the Equatorial upwelling region with predominantly maritime aerosols.
- Comparison and in-water radiometers (e.g. WiSPER, TACCS, SATLANTIC HyperPRO II) under in open ocean and turbid coastal with predominantly continental aerosols.
- Comparison of above-water fixed and sun glint reduction (using automated tracking system) methods.
- Comparison of in-water methods including underwater profiling from winch, freefall and at fixed-depths.
- Different processing schemes are applied to the data from these systems.
- Quantification of the source of uncertainty in the radiometric measurements
- Antoine, D., d’Ortenzio, F., Hooker, S. B., Becu, G., Gentili, B., Tailliez, D., et al. Assessment of uncertainty in the ocean reflectance determined by three satellite ocean color sensors (MERIS, SeaWiFS and MODIS-A) at an offshore site in the Mediterranean Sea (BOUSSOLE project). Journal of Geophysical Research-Oceans, 113, C07013, 2008.
- Brewin, R. J. W., Dall’Olmo, G. Evaluating ocean-colour data using underway optical sampling along the Atlantic Meridional Transect (AMT): Chlorophyll-a concentration. Presentation at the Ocean Optics XXII conference, Portland Maine, US, 26-31 Oct 2014, 2014
- D’Alimonte, D., Zibordi, G., Kajiyama, T., and Cunha, J. C.: A Monte Carlo code for high spatial resolution ocean color simulations, Appl. Optics, 49, 4936–4950, 2010.
- Deschamps, P.-Y., Fougnie, B., Frouin, R., Lecomte, P., and Verwaerde, C.: SIMBAD: a field radiometer for satellite ocean-color validation, Appl. Optics, 43, 4055–4069, 2004.
- Dall’Olmo G., Boss E., Behrenfeld M. and Westberry T. Particulate optical scattering coefficients along an Atlantic Meridional Transect. Opt. Express 20, 21532-21551, 2012.
- Fougnie, B., Frouin, R., Lecomte, P., and Deschamps, P. Y.: Reduction of skylight reflection effects in the above-water measurement of diffuse marine reflectance, Appl. Optics, 38, 3844–3856, 1999.
- Hooker, S. B., Lazin, G., Zibordi, G., and McClean, S.: An evaluation of above- and in-water methods for determining water leaving radiances, J. Atmos. Ocean. Tech., 19, 486–515, 2002a.
- Hooker, S. B., McLean, S., Small, M., Lazin, G., Zibordi, G., and Brown, J.: The Seventh SeaWiFS Intercalibration Round-Robin Experiment (SIRREX-7), March 1999. SeaWiFS Report NASA/TM-2001- 206892, vol. 17, edited by: Hooker, S. B. and Firestone, E. R., NASA Goddard Space Flight Center, Greenbelt, Maryland, 2002b.
- Hooker, S. B., Zibordi, G., Berthon, J.-F., and Brown, J.W.: Above-Water Radiometry in shallow coastal waters, Appl. Optics, 43, 4254–4268, 2004.
- Hooker, S. B. and Zibordi, G.: Platform perturbation in Above-Water Radiometry, Appl. Optics, 44, 553–567, 2005.
- Mobley, C. D.: Estimation of the remote sensing reflectance from above–water methods, Appl. Optics, 38, 7442–7455, 1999.
- Moore, G. F., Icely, J. D., and Kratzer, S.: Field Inter-comparison and validation of in-water radiometer and sun photometers for MERIS validation, Proceedings of the ESA Living Planet Symposium, Special Publication SP-686, 2010.
- O’Reilly, J. E., Maritorena, S., Mitchell, B. G., Siegel, D. A., Carder, K. L., Garver, S. A., et al. Ocean color chlorophyll algorithms for SeaWiFS. Journal of Geophysical Research-Oceans, 103, 24937–24953, 1998
- Ruddick, K., De Cauwer, V., Park, Y., and Moore, G.: Seaborne measurements of near infrared water-leaving reflectance – the similarity spectrum for turbid waters, Limnol. Oceanogr., 51, 1167–1179, 2006.
- Tilstone GH, Moore GF, Sorensen K, Ruttgers R, Viskum PY, Martinez-Vicente V, Ruddick KG. (2003). Regional validation of MERIS Chl products in North Sea coastal waters: MAVT inter-calibration results. Proceedings from ENVISAT MAVT Conference, 20 – 24 October 2003, Frascatti, Italy. European Space Agency. http://envisat.esa.int/workshops/mavt_2003/MAVT-2003_803_REVAMP_Intercal_report_final.pdf
- Zibordi, G., Hooker, S. B., Berthon, J.-F., and D’Alimonte, D.: Autonomous above–water radiance measurement from an offshore platform: A field assessment, J. Atmos. Ocean. Tech., 19, 808–819, 2002.
- Zibordi, G., D’Alimonte, D., and Berthon, J.-F.: An evaluation of depth resolution requirements for optical profiling in coastal waters, J. Atmos. Ocean. Tech., 21, 1059–1073, 2004a.
- Zibordi, G., Melin, F., Hooker, S. B., D’Alimonte, D., and Holben, B.: An autonomous above-water system for the validation of ocean color radiance data, IEEE Trans. Geosc. Rem. Sens., 42, 401–415, 2004b.
- Zibordi, G., Melin, F., and Berthon, J.-F.: Comparison of SeaWiFS, MODIS and MERIS radiometric products at a coastal site, Geophys Res. Lett., 33, L06617, doi:10.1029/2006GL025778, 2006.
- Zibordi, G., Berthon, J.-F., and D’Alimonte, D.: An evaluation of radiometric products fixed-depth and continuous in-water profile data from a coastal site, J. Atmos. Ocean. Tech., 26, 91–186, 2009a.
- Zibordi, G., Berthon, J.-F., M´elin, F., D’Alimonte, D., and Kaitala, S.: Validation of satellite ocean color primary products at optically complex coastal sites: Northern Adriatic Sea, Northern Baltic Proper and Gulf of Finland, Remote Sens. Environ., 113, 2574–2591, 2009b.
- Zibordi, G., Holben, B., Slutsker, I., Giles, D., D’Alimonte, D, Melin, F., Berthon, J.-F., Vandemark, D., Feng, H., Schuster, G., Fabbri, B.E., Kaitala, S., and Sepp¨al¨a, J.: AERONET-OC: a network for the validation of Ocean Color primary radiometric products, J. Atmos. Ocean. Tech., 26, 1634–1651, 2009c.
- Zibordi, G., Ruddick, K., Ansko, I., Moore, G. F., Kratzer, S., Icely, J. D., Reinhart, A. In situ determination of the remote sensing reflectance: an inter-comparison, Ocean Sci., 8, 567–586, 2012