Copernicus is a European system for monitoring the Earth. It includes earth observation satellites (notably the Sentinel series developed by ESA), ground-based measurements and services to processes data to provide users with reliable and up-to-date information through a set of Copernicus Services related to environmental and security issues.
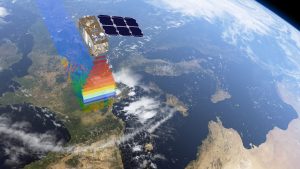
Colour vision for Copernicus © ESA/ATG medialab
These include:
• Copernicus Marine Environment Monitoring Service (CMEMS),
• Copernicus Global Land Service (CGLS),
• Copernicus Atmospheric Monitoring Service (CAMS),
• Copernicus Emergency Management Service (CEMS),
• Copernicus Climate Change Service (C3S).
They will provide critical information in near-real time to support a wide range of applications, including environment protection, management of urban areas, regional and local planning, agriculture, forestry, fisheries, health, transport, climate change, sustainable development, civil protection and tourism. Copernicus satellite missions are designed to serve all Copernicus Services by providing systematic measurements of Earth’s oceans, land, ice and atmosphere to monitor and understand large-scale global dynamics.
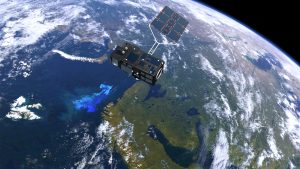
Revealing the colour of ocean life © Copyright ESA/ATG medialab
The primary users of Copernicus services are policymakers and public authorities who need the information to develop environmental legislation and policies or to take critical decisions in the event of an emergency, such as a natural disaster or a humanitarian crisis. The Copernicus programme is coordinated and managed by the European Commission. The development of the observation infrastructure is performed under the aegis of the European Space Agency for the space component and of the European Environment Agency and the Member States for the in situ component.
The societal Benefits of Ocean Colour Radiometry (OCR) are well articulated (e.g. IOCCG Report, “Why Ocean Colour? The Societal Benefits of Ocean colour Radiometry” (2008), CEOS OCR-VC, IOCCG) and include management of the marine ecosystem, role of the ocean ecosystem in climate change, aquaculture, fisheries, coastal zone water quality, mapping and monitoring harmful algal blooms. Consequently, Copernicus has developed two relevant satellite families (Sentinel-2 and Sentinel-3) that carry two complementary payload instruments that can measure ocean colour to support the CMEMS service. Sentinel-2 and Sentinel- 3 will serve the broad scope of Copernicus Service application areas using a unique suite of instruments and data products to allow European environmental policies to be administered with confidence.
Sentinel-3 (S3) is a twin-satellite constellation operating in a sun-synchronous near polar orbit and is the most complex of all the Sentinel missions in view of the single-satellite multi-instrument configuration (Donlon et al, 2012). S3 is the core operational mission for the CMEMS service making a contribution to ~70% of all CMEMS user products.
Sentinel-3 is an unprecedented step forward for operational oceanography and for CMEMS providing continuous and essential observations for ocean analysis and forecasting both for physics and biogeochemistry. In order to satisfy the large coverage and high revisit requirements, the Sentinel-3 mission is designed as a constellation of two identical satellites, Sentinel-3A (S3A) and Sentinel-3B (S3B). Both S3A and S3B are designed for a nominal lifetime of ~7 years, with consumable for up to 12 years. S3A was launched on 16 Feb 2016 and S3B is expected ~18 months later.
Each Sentinel-3 satellite is developed, launched and commissioned by ESA. EUMETSAT will then operate each satellite and take responsibility for the Marine data production for the mission. Both ESA and EUMETSAT will contribute to the maintenance of the mission including calibration and validation aspects.
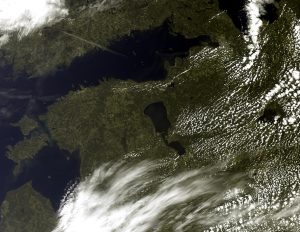
Sentinel-3 picture. Estonia on 14 Jun 2016.
The main objectives of the mission is to provide a sustained operational capability to measure sea- and land surface temperature, sea surface topography, sea ice freeboard/thickness, and ocean- and land surface colour/reflectance with excellent accuracy and reliability in support of Copernicus Services.
Sentinel-3 satellite carries as part of its payload an Ocean and Land Colour Instrument (OLCI), a spectrometer imaging in pushbroom mode with an across-track electronic scan, building on the heritage of ENVISAT MERIS.
The design aim is for an instrument capable of measuring ocean and land colour with high absolute (relative) accuracy. OLCI will provide a native 300 m resolution 21-band data product for all illuminated surfaces across a swath of 1270 km. The OLCI instrument is tilted 12.6° to the west of the sub-satellite point in an effort to mitigate sun-glint. The minimum revisit time at the equator is ~2 days using two satellites. On-board calibration of the OLCI instrument follows the same approach as MERIS using solar diffusers [RD-12]. OLCI will systematically acquire imagery at a native resolution of 300 m over all Earth surfaces illuminated by the sun.
The Sentinel-2 mission (Fletcher, K. (Ed.), (2012), Drusch, M., et al, (2012), ESA Sentinel web site is designed to ensure the continuity of services that rely on multispectral high-resolution optical observations over global terrestrial surfaces and coastal zones.
The mission objectives are to provide systematic acquisitions of high-resolution multispectral imagery with a high revisit frequency, to ensure the continuity of multispectral imagery provided by the SPOT series of satellites, and to provide observations for the next generation of operational products such as land-cover maps, land-use change detection maps and geophysical variables. Sentinel-2 will contribute directly to land monitoring, emergency response and security services.
Sentinel-2 is also a two-satellite constellation operating in a day sun-synchronous orbit at 786 km with a local time at descending node of 10:30 am. Each satellite flies on the same orbital plane separated by 180°. A 5-day repeat cycle at the equator using the 2-satellite constellation is achieved. Each Sentinel-2 satellite will be developed, launched, commissioned and operated by ESA. Sentinel-2A launched in June 2015 from the ESA Space Port, Kourou French Guyana and a second Sentinel-2B is expected in 2017.
The Sentinel-2 mission Multi-Spectral Imager (MSI) is a push-broom instrument and has a wide swath of 290 km with 13 spectral bands (VIS, NIR and SWIR), at 10, 20 and 60 m spatial resolution.
MSI uses a Three-Mirror Anastigmat (TMA) telescope together with two focal planes (one for visible and near infrared channels and a second for short-wave infrared channels). Two distinct arrays of 12 detectors mounted on each focal plane covering VNIR and SWIR channels respectively. The 12 detectors on each focal plane are in a staggered configuration to cover the entire field of view. MSI uses a dichroic VNIR/SWIR beam splitter and stripe filters mounted on top of the detectors provide spectral separation in the various bands. The Sentinel-2 MSI represents the state of the art in satellite multi-spectral imaging capability.
The baseline for full Sentinel-2 MSI operations includes the systematic acquisition of:
• All land surfaces (-56° and +84° latitude);
• Major and EU islands (greater than 100 km² size);
• Coastal regions extending 20 km from the coast;
• Inland waters, Mediterranean Sea and all closed seas;
• Ocean calibration and validations sites including MOBY and Boussole;
Significant acquisitions over some coastal areas are expected when the orbit ground track is aligned roughly parallel to the coastline because the entire swath is acquired if just one pixel falls over the land/coastal boundary. Sentinel-2 MSI will thus make a significant contribution to satellite OCR.
Calibration and Validation of Satellite Ocean Colour Sensors
Satellite ocean colour sensors are designed to retrieve the spectral distribution of upwelling radiance just above the sea surface (the water-leaving radiance) that is then used to estimate a number of geophysical parameters through the application of specific biooptical algorithms. Accurate radiometric calibration and characterization of the individual satellite sensors is the most critical component toward achieving the goal of consistent, long-term multi-mission Ocean Colour products [AD-1]. Pre-launch calibration and characterization data are essential for understanding the instrument measurement uncertainties, to correct for measurement artefacts and to have a point of reference for monitoring instrument changes on orbit.
Once on-orbit, the uncertainty characteristics of: (a) the satellite instruments established during pre-launch laboratory calibration and characterisation activities and (b) the end-to-end geophysical measurement retrieval process can only be assessed via independent calibration and validation activities.
The Committee for Earth Observation Satellites (CEOS) [URL-4][URL-6] define
Calibration as “the process of quantitatively defining a system’s responses to known, controlled signal inputs”.
Validation, on the other hand, is “the process of assessing, by independent means, the quality [uncertainty] of the data products derived from those system outputs”. Validation is a core component of a satellite mission (and should be planned for accordingly) starting at the moment satellite instrument data begin to flow until the end of the mission.
Adjustment is “set of operations carried out on a measuring system so that it provides prescribed indications corresponding to given values of a quantity to be measured”. Adjustment of a measuring system should not be confused with calibration, which is a prerequisite for adjustment [JCGM 200:2012].
Ground Measurements
Therefore ground measurements are essential to the Sentinel-2 MSI and Sentinel-3 OLCI OCR.
Ground measurements collected at times close to the satellite overpass are fundamental for:
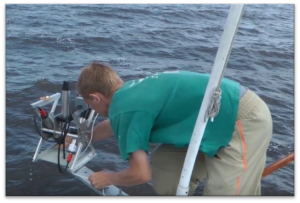
Ground measurements are essential to the Sentinel-2 MSI and Sentinel-3 OLCI OCR © TO
- Vicarious adjustment of the satellite OCR L2 products;
- Continuous assessment of OCR quality (i.e., validation of normalized water leaving radiance or the equivalent remote sensing reflectance);
- Validation of derived satellite ocean colour products (e.g., chlorophyll-a concentration) and;
- Development and verification of the bio-optical algorithms required for generating derived products (independent of any specific satellite mission).
Quality Assurance of Data
The central position of ground-based data for satellite OCR requires action to assure their quality, preservation and accessibility. If ground-based measurements are to be credibly used for satellite validation activities (particularly for assessments of climate data record stability [e.g. RD-6]) then they must be obtained contemporaneously and co-located with satellite measurements and be accurate and precise.
As noted in 1995 at the 20th Conference Generale des Poids et Mesures [RD7], a recommendation was made that:
‘‘those responsible for studies of Earth resources, the environment, human wellbeing and related issues ensure that measurements made within their programs are in terms of well-characterized SI units so that they are reliable in the long term, are comparable world-wide and are linked to other areas of science and technology through the world’s measurement system established and maintained under the Convention du Metre’’.
This lays the foundation to relate satellite measurements to Systeme International d’Unites (SI) and collecting Climate Data Records measurements from different satellite and ground-based sources over a period of time, in a way that they can be combined in a meaningful manner [RD-5].
Fiducial Reference Measurements
In order to achieve that, the concept of Fiducial Reference Measurements (FRM) has been established by the Sentinel-3 Validation Team (S3VT) as:
“The suite of independent ground measurements that provide the maximum Return On Investment (ROI) for a satellite mission by delivering, to users, the required confidence in data products, in the form of independent validation results and satellite measurement uncertainty estimation, over the entire end-to-end duration of a satellite mission.”
The defining mandatory characteristics for FRM are:
- FRM measurements have documented SI traceability (eg. via round-robin intercalibration of instruments) using metrology standards (eg. [RD-4], [AD-2]).
- FRM measurements are independent from the satellite geophysical retrieval process [noting the exception of L2 product vicarious adjustment that fundamentally depends on FRM ground based measurements],
- An uncertainty budget for all FRM instruments and derived measurements is available and maintained (eg. [RD-5]).
- FRM measurement protocols and community-wide management practices (measurement, processing, archive, documents etc.) are defined, published openly and adhered to by FRM instrument deployments.
- FRM measurements are openly and freely available for independent scrutiny.
FRM are required to determine via independent validation activities the in-orbit uncertainty characteristics of satellite geophysical measurements and this is what the FRM4SOC project is going to establish.
The aim of the FRM4SOC project is:
To establish and maintain SI traceability of Fiducial Reference Measurements (FRM) for satellite ocean colour radiometry (OCR).
The Remote Sensing Reflectance
The primary radiometric parameter deduced from satellite measurements is the remote sensing reflectance, which is also called “water-leaving radiance reflectance” when multiplied by π. This is the reflectance at sea level obtained by dividing the upwelling radiance, leaving the water in the direction of the satellite sensor, by the downwelling irradiance, illuminating the water from the sun and sky. A precise definition of these terms can be found in the “Light and Radiometry” section of the Ocean Optics Web Book.
The remote sensing reflectance and underlying upwelling radiance and downwelling irradiance are all spectral quantities, meaning that they are measured at different wavelengths. The human perception of colour is based on measurement at three wavelengths, but satellite and ship-based radiometers can measure many more wavelengths, e.g. 21 for OLCI.
There are a range of different methods for measuring both the upwelling radiance and the downwelling irradiance.
Measuring the Upwelling Radiance
The upwelling radiance can be measured by
a) pointing a radiometer at the sea from a ship or a fixed platform, a few metres above the sea surface;
b) positioning a radiometer vertically downwards from a ship or floating buoy just beneath (a few mm or cm) the sea surface;
c) positioning downward-pointing radiometers fixed to a underwater structure at multiple depths (typically a few metres) below the sea surface and extrapolating these measurements to the sea surface, or;
d) measuring continuous vertical profiles with a downward-pointing radiometer, fixed on a profiling float or buoy or a ship winch, and extrapolating these measurements to the sea surface.
Measuring the Downwelling Irradiance
The downwelling irradiance can be measured by
a) a radiometer, mounted abovewater on a ship, fixed platform or buoy, pointing vertically upwards;
b) a sunphotometer, mounted abovewater on a fixed platform, measuring the direct sun radiance and using an atmospheric optical model to deduce full sky downwelling irradiance;
c) an upward-pointing radiometer deployed just beneath the sea surface, or; d) upward-pointing radiometers deployed just beneath the sea surface either at fixed depths or vertically profiling a continuous range of depths.
Diversity of Methods
There is, thus, a high diversity of methods used, both in the basic measurement principle, the choice of radiometer, the type of deployment platform (offshore platform, buoy, ship, etc.), and the details of the data processing and associated approximations and corrections. Each method has associated advantages/disadvantages for both data quality (some methods may perform better, for example, in turbid/clear waters or for longer/shorter wavelengths) and for data quantity (autononmous vs supervised systems) as well as having different logistical requirements and hence cost.
The objective of FRM4SOC is
To establish the measurement uncertainty of each method and hence establish which measurements can be considered as Fiducial Reference Measurements.
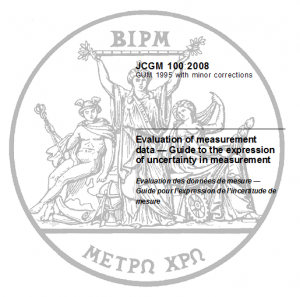
All measurements are imperfect and have errors that can be of a random nature (ie. noise on charge couple device detectors) or of a systematic nature (e.g. incorrect characterization of spectral response function). Increasing the number of measurements can reduce random errors through computation of statistical averages that reduce the random uncertainty component.
Systematic errors can only be corrected using an appropriate correction factor. Clearly all recognised systematic errors must be corrected and random errors reduced if a reliable and accurate measurement is to be obtained from any instrument – either on the ground or in space.
Uncertainties arise due to many aspects that can be generally grouped into the following primary categories:
• Instrument measurement uncertainty: those relating to instrument hardware,
• Retrieval/algorithm uncertainty: those relating to derived quantities,
• Application uncertainty: those relating to a specific application,
• Unknown: those uncertainties that are “unknown”.
For each category standard practice requires an uncertainty budget to be derived including all aspects leading to a quantification of a root-sum-square (RSS) estimate of uncertainty. This is a challenging exercise but nevertheless, for climate and satellite validation activities, it is a requirement.
Establishing an uncertainty budget for FRM is a fundamental step that drives a better understanding of the various components of FRM uncertainty: quite often an instrument engineer will learn much about an instrument and its fitness for purpose by attempting the derivation of a full instrument uncertainty budget – potentially leading to innovation and improvement in design.
But the real driver is to remember that, if reliable and well-defined uncertainties can be provided with each FRM field radiometer, then these measurements can uniquely provide an SI traceable measurement on a per-measurement basis when matched to satellite measurements – without the need for many observations to reduce the random error.
What means tracebility to SI?
Metrology, the science of measurement, is practised at the International Bureau of Weights and Measures (BIPM) and the National Metrology Institutes (NMIs) who research physical standards and constants, and methods to realise and disseminate units including the International System of Units (SI; BIPM, 2014).
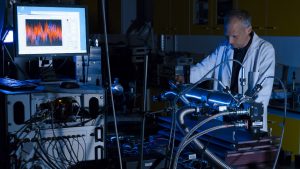
NPL Cryogenic Radiometer – Primary Standard for Optical Measurement © NPL Management Ltd. Photo: Courtesy of NPL.
SI provides the foundation for measurement around seven base units and a system of coherent derived units. The SI units must be stable over centuries. They must also be uniform worldwide and independent of the method used to realise the unit (Woolliams et al., 2016).
The metrological community achieves this through three technical concepts: traceability, uncertainty analysis and comparison. Metrological traceability is a property of a measurement that relates the measured value to a stated metrological reference through an unbroken chain of calibrations or comparisons. Uncertainty analysis is the review of all sources of uncertainty and the propagation of that uncertainty through the traceability chain. The traceability and uncertainty analysis at the NMIs is rigorously audited through the Mutual Recognition Arrangement, which also involves regular formal international comparisons (Woolliams et al., 2016).
For ocean colour measurements, whether they are made by satellite or in situ ocean colour radiometers, traceability to SI is achieved through an unbroken series of calibrations back to the primary standard for optical radiation: the NPL cryogenic radiometer. This traceability chain is visualized for FRM4SOC in the figure below.
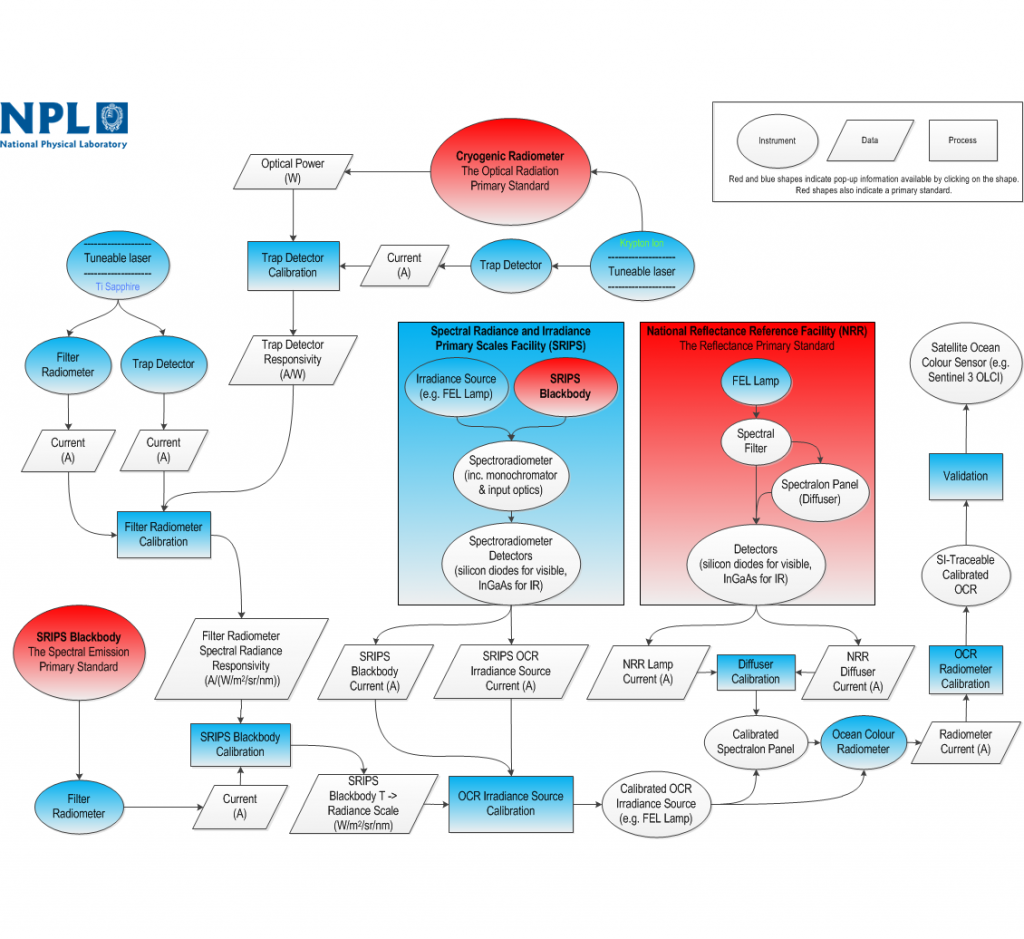
SI-tracebility Chain Diagram
Importance of SI-tracebility
SI-traceability is important for satellite ocean colour measurements and validation because it provides a meaningful way of:
- Making the measurements truly fiducial by having the same standardised reference (SI);
- Combining the measurements from different ocean colour sensors or at least making them comparable;
- Quantifying the stability of ocean colour sensors and their data and making these reliable over the long-term, which is essential for monitoring the effects of, for example, climate change. This includes helping to facilitate the creation of reliable climate data records from ocean colour and the associated in situ validation data bases.
- Determining quantitatively the radiometric differences between different sensors and their uncertainties.
The development of the Quality Assurance Framework for Earth Observation (QA4EO) by the Committee on Earth Observation Satellites (CEOS) was an initial step to encourage the adoption of metrological principles, including SI traceability, in the EO community. These principles are starting to become more widely applied in EO measurements. A good example is the Fiducial Reference Measurement (FRM) series of activities pioneered by ESA, which includes this project on satellite ocean colour.
References
BIPM (2014). SI Brochure: The International System of Units (SI) (8th Edition).
Woolliams, E.R., Mittaz, J.P.D., Merchant, C.J., Dilo, A. and Fox, N.P., 2016. Uncertainty and Correlation in Level 1 and Level 2 Products – A Metrologist’s View. The European Space Agency Living Planet Symposium 2016, Prague, Czech Republic, 9-13 May 2016.